Mesenchymal stem cells as delivery vectors for anti-tumor therapy
Introduction
Cancer remains a major public health problem worldwide and it is one of the leading causes of mortality and morbidity (1). The principal approaches of cancer therapy include surgical treatment, chemotherapy and radiotherapy. However, despite improvements in therapeutic methods, many tumors remain to have poor prognosis after traditional therapy, due to relapse and metastasis. Biological therapy has been noted as a novel strategy to various tumor diseases, especially for the relapsed patients. One of the major challenges of cancer biological therapy lies in the inefficient delivery of therapeutic agents to the tumor sites due the relapsed or metastatic tumors are often smaller and directly inaccessible. It has recently been shown that mesenchymal stem cells (MSCs) are able to migrate specifically to and integrate into tumor stroma and track microscopic metastasis when administered by intravenous in vivo (2,3), and this property can be used to deliver anti-cancer agents for tumor treatment. However, the interaction between tumors and MSCs remains controversial. This review focuses on the application of MSCs for targeted delivery of anti-cancer agents to tumors, and on the interaction between tumors and MSCs.
Sources and characteristics of MSCs
Human MSCs are a rare stromal cell population that resides primarily in adult bone marrow (BM), and considered as non-hematopoietic multipotent stem-like cells that have self-renewing potential, capable of differentiating into lineages of mesenchymal or non-mesenchymal tissues, including osteoblasts, chondrocytes, adipocytes, myocytes, tendon and neuronal cells, etc. (4,5). Since Pittenger et al. demonstrated the successful isolation of multipotent MSCs from human BM, it has become the principal source to obtain MSCs (4). However, only a small percentage of the total number of BM populating cells is MSCs (0.01-0.001% of mononuclear cells isolated by ficoll density gradient); moreover, the invasive isolation procedure and the decline in MSC characteristics with donor’s age also restrict its clinical application (6). In addition, adipose tissue has recently proven to serve as an alternative source of MSCs. Although it’s abundant in nature, an invasion isolation procedure is still required to collect the tissue (7). MSCs obtained from the Wharton’s Jelly (WJ) of umbilical cords (UC), UC blood or placenta have gained much attention over the last years since they can be easily isolated, without any ethical concerns, from tissues which are discarded after birth (8). Moreover, those extra-embryonic tissue-derived MSCs have little or low immunogenicity due to the lack of expression of co-stimulatory molecules, possess a broader multipotent plasticity and proliferate faster than adult MSCs (9). Accumulating evidences demonstrate that extra-embryonic tissue-derived MSCs are an attractive stem cell source for clinical applications (10-12).
There are no specific cell markers that can be used to categorically define human MSCs, and current characterization based on combination criteria of adherence to plastic, expression of CD73, CD90 and CD105 but lack of haematopoietic cell markers such as CD34, CD45, CD19, CD11b, HLA-DR on the cell surface and multi-differentiation potential into osteoblasts, adipocytes and chondroblasts in vitro (13). Moreover, MSCs can be easily engineered with viral vectors and provide long-term gene expression without affecting their MSC phenotype (14,15). Interestingly, MSCs have the ability to accumulate at the site of damaged tissue, inflammatory and cancer sites when administered intravenously in vivo (15-17). Hence, these characteristics make MSCs to be used for regenerative therapy and as cellular vehicles for the delivery of anti-cancer agents specifically to tumors. This targeted therapy can facilitate the anti-cancer agents to accumulate at local tumor sites and reach effective concentrations, especially in favor of the deep-seated solid tumors and the micro-metastatic tumors that are not often accessible directly.
MSCs as delivery vectors for cancer therapy
More and more investigators have explored MSCs in delivering anti-cancer agents due to its ability of tumor tropism. The anti-cancer agents carried by MSCs mainly include retroviral or adenoviral vectors expressing tumor-suppressed gene, oncolytic viruses and drug-loaded polymeric nanoparticles (Table 1).
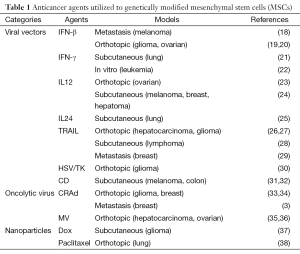
Full table
Viral vectors engineered MSCs
Human MSCs have been engineered to provide targeted delivery of anti-cancer agents by adenovirus or retrovirus transduction. The earliest application of MSCs to targeted cancer therapy was direct delivery of interferon β (IFN-β) gene (18). MSCs were transduced with an adenoviral vector carrying the IFN-β gene, followed by i.v. injection into melanoma xenografts mice. The results showed that treatment with IFN-β-MSCs significantly reduced tumor growth and prolonged survival of tumor-bearing mice (18). Based on this discovery, more researchers have since studied the therapeutic efficacy of genetically modified MSCs from various tissues and their ability to act as cellular vehicles. In addition to IFN-β gene (19,20), other tumor-suppressed genes including IFN-γ (21,22), IL-12 (23,24), IL-24 (25) and tumor necrosis factor-related apoptosis inducing ligand (TRAIL) (26-29) have been utilized to engineer MSCs, and could be produced locally in the tumor microenvironment to treat a wide range of cancers by inhibiting tumor cells growth or inducing apoptosis, as described in Table 1.
MSCs have also been used to serve as vehicles for suicide gene/enzyme prodrug systems, which can convert non-toxic prodrug into toxic anti-metabolites and kill tumor cells selectively. Uchibori et al. discovered that injection of MSCs producing herpes simplex virus thymidine kinase (HSV-TK) retroviral vectors in the proximity of tumors with administration of prodrug GCV suppressed tumor growth through bystander-mediated tumor cell killing in vitro and in vivo (30). In other two different studies, MSCs expressed cytosine deaminase (CD) treatment resulted in therapeutic cell homing into tumor sites and mediated melanoma (31) and colon cancer (32) growth inhibition. Nevertheless, these approaches are limited by the toxicity to the MSCs.
Oncolytic viruses loaded MSCs
In addition to the delivery of tumor-suppressed genes, MSCs have been employed as carriers and amplifiers to deliver oncolytic viruses to tumor sites, leading to destroy of tumor cells by viral replication and spread surrounding tumor tissues. At present, the therapeutic efficacy of MSCs engineered by conditionally replicative adenovirus (CRAd) and oncolytic measles virus (MV) has been evaluated in experimental and clinical trials. This targeted therapy has been shown to improve survival and suppress metastasis in a human glioma model (33), melanoma and breast cancer model (34), and a lung metastasis model (3) using oncolytic CRAd. Besides, attenuated MV-infected MSCs cell delivery system could also inhibit liver cancer growth (35), and prolong survival of animals bearing ovarian cancer (36), eluding the presence of immunity against MV in vivo.
Drug-loaded nanoparticles engineered MSCs
Recently, Li et al. have demonstrated that silica nanorattle-doxorubicin anchored MSCs could be capable of accumulating in tumors and slowly releasing the drug with high cell viability. The increased and prolonged doxorubicin intratumoral distribution further contributed to significantly enhanced tumor cell apoptosis in human glioma xenografts (37). Similarly, MSCs loaded with paclitaxel containing nanoparticles have showed active tumor-tropism and released paclitaxel at tumor site locally in a mouse orthotopic lung tumor model (38). This strategy has the potential to be developed as a targeted tumor therapy with high efficiency and low systematic toxicity. The therapeutic benefit of this strategy should be further examined in orthotopic and metastatic tumor models.
Interaction between cancer and MSCs
The ability of MSCs to accumulate at tumor sites makes them extremely attractive for directed cancer therapy. However, MSCs are not simple inert vectors, the possible direct effect of MSCs on tumor growth should not be neglected. At present, no consensus exists on the effects of MSCs may have on the host. For example, Qiao’s study proved that MSCs inhibit growth of breast cancer cells via depression of Wnt signaling (39), while Karnoub suggested the MSCs promote tumor development in a subcutaneous breast tumor model (40). Additionally, no positive or negative effect of MSCs was revealed in a glioblastoma model (26) and in a Non-Hodgkin’s lymphoma (28). The diverse effects of MSCs on tumor growth among these studies might be due to the different source of MSCs, the different animal models, and the route of MSCs administration.
Furthermore, it is known that MSCs have profound immunosuppressive effects. Ling et al. demonstrated that MSCs could create an immunosuppressive microenvironment and thus promote tumor growth using humanize murine MSCs by incorporating the human IDO gene into MSCs derived from mice lacking the Nos2 gene (iNOS−/−) (41). This strategy has got rid of species variation and represents the immune state in human closely. However, immunologic deficiency mice were employed in the general studies about MSCs used in cancer therapy, which cannot reflect immunosuppressive effect of MSCs on the tumor. Besides, MSCs could enhance tumor growth by some other mechanisms, such as the VEGF pathway (42), and chemokine CCL5 secretion (40).
As clinical applications of MSCs develop further, the assessment of the long-term fate of therapeutic MSCs is critical, and we should seek to develop a system allowing control over the growth and survival of MSCs post tumor treatment. In the oncolytic viruses-loaded MSCs delivery strategy, oncolytic viruses replicated in MSCs and destroyed them eventually and eliminate the tumor promotion effects (3,33-36). Martinez-Quintanilla et al. have developed an efficient stem cell based therapeutic strategy that MSCs engineered to co-express HSV-TK and s-TRAIL, simultaneously allowed killing of glioblastoma multiforme cells and assessment and eradication of MSCs post treatment (43). Moreover, Ramos et al. have also described a suicide system based on an inducible caspase-9 (iCasp9) protein to eliminate MSCs used in therapy (44).
Conclusions
MSCs are emerging as effective vectors for targeted anticancer treatment. As this promising therapy is to be translated into clinical application, there are many basic questions to be resolved, such as the optimal timing of delivery, the number of cells injected, and the effect of MSCs on tumors. So, more studies about MSCs as vectors for anti-cancer therapy are urgently needed to push this strategy to the clinic.
Acknowledgements
None.
Footnote
Conflicts of Interest: The authors have no conflicts of interest to declare.
References
- Siegel R, Naishadham D, Jemal A. Cancer statistics, 2013. CA Cancer J Clin 2013;63:11-30. [PubMed]
- Mohr A, Albarenque SM, Deedigan L, et al. Targeting of XIAP combined with systemic mesenchymal stem cell-mediated delivery of sTRAIL ligand inhibits metastatic growth of pancreatic carcinoma cells. Stem Cells 2010;28:2109-20. [PubMed]
- Stoff-Khalili MA, Rivera AA, Mathis JM, et al. Mesenchymal stem cells as a vehicle for targeted delivery of CRAds to lung metastases of breast carcinoma. Breast Cancer Res Treat 2007;105:157-67. [PubMed]
- Pittenger MF, Mackay AM, Beck SC, et al. Multilineage potential of adult human mesenchymal stem cells. Science 1999;284:143-7. [PubMed]
- Giordano A, Galderisi U, Marino IR. From the laboratory bench to the patient's bedside: an update on clinical trials with mesenchymal stem cells. J Cell Physiol 2007;211:27-35. [PubMed]
- Batsali AK, Kastrinaki MC, Papadaki HA, et al. Mesenchymal stem cells derived from Wharton's Jelly of the umbilical cord: biological properties and emerging clinical applications. Curr Stem Cell Res Ther 2013;8:144-55. [PubMed]
- Bunnell BA, Flaat M, Gagliardi C, et al. Adipose-derived stem cells: isolation, expansion and differentiation. Methods 2008;45:115-20. [PubMed]
- Kim DW, Staples M, Shinozuka K, et al. Wharton's Jelly-Derived Mesenchymal Stem Cells: Phenotypic Characterization and Optimizing Their Therapeutic Potential for Clinical Applications. Int J Mol Sci 2013;14:11692-712. [PubMed]
- Marcus AJ, Woodbury D. Fetal stem cells from extra-embryonic tissues: do not discard. J Cell Mol Med 2008;12:730-42. [PubMed]
- Anzalone R, Lo Iacono M, Corrao S, et al. New emerging potentials for human Wharton's jelly mesenchymal stem cells: immunological features and hepatocyte-like differentiative capacity. Stem Cells Dev 2010;19:423-38. [PubMed]
- Fong CY, Subramanian A, Gauthaman K, et al. Human umbilical cord Wharton's jelly stem cells undergo enhanced chondrogenic differentiation when grown on nanofibrous scaffolds and in a sequential two-stage culture medium environment. Stem Cell Rev 2012;8:195-209. [PubMed]
- Taghizadeh RR, Cetrulo KJ, Cetrulo CL. Wharton's Jelly stem cells: future clinical applications. Placenta 2011;32 Suppl 4:S311-5. [PubMed]
- Dominici M, Le Blanc K, Mueller I, et al. Minimal criteria for defining multipotent mesenchymal stromal cells. The International Society for Cellular Therapy position statement. Cytotherapy 2006;8:315-7. [PubMed]
- Lee K, Majumdar MK, Buyaner D, et al. Human mesenchymal stem cells maintain transgene expression during expansion and differentiation. Mol Ther 2001;3:857-66. [PubMed]
- Ozawa K, Sato K, Oh I, et al. Cell and gene therapy using mesenchymal stem cells (MSCs). J Autoimmun 2008;30:121-7. [PubMed]
- Sohni A, Verfaillie CM. Mesenchymal stem cells migration homing and tracking. Stem Cells Int 2013;2013:130763.
- Kidd S, Spaeth E, Dembinski JL, et al. Direct evidence of mesenchymal stem cell tropism for tumor and wounding microenvironments using in vivo bioluminescent imaging. Stem Cells 2009;27:2614-23. [PubMed]
- Studeny M, Marini FC, Champlin RE, et al. Bone marrow-derived mesenchymal stem cells as vehicles for interferon-beta delivery into tumors. Cancer Res 2002;62:3603-8. [PubMed]
- Dembinski JL, Wilson SM, Spaeth EL, et al. Tumor stroma engraftment of gene-modified mesenchymal stem cells as anti-tumor therapy against ovarian cancer. Cytotherapy 2013;15:20-32. [PubMed]
- Nakamizo A, Marini F, Amano T, et al. Human bone marrow-derived mesenchymal stem cells in the treatment of gliomas. Cancer Res 2005;65:3307-18. [PubMed]
- Yang X, Du J, Xu X, et al. IFN-γ-secreting-mesenchymal stem cells exert an antitumor effect in vivo via the TRAIL pathway. J Immunol Res 2014;2014:318098.
- Li X, Lu Y, Huang W, et al. In vitro effect of adenovirus-mediated human Gamma Interferon gene transfer into human mesenchymal stem cells for chronic myelogenous leukemia. Hematol Oncol 2006;24:151-8. [PubMed]
- Zhao WH, Cheng JX, Shi PF, et al. Human umbilical cord mesenchymal stem cells with adenovirus-mediated interleukin 12 gene transduction inhibits the growth of ovarian carcinoma cells both in vitro and in vivo. Nan Fang Yi Ke Da Xue Xue Bao 2011;31:903-7. [PubMed]
- Chen X, Lin X, Zhao J, et al. A tumor-selective biotherapy with prolonged impact on established metastases based on cytokine gene-engineered MSCs. Mol Ther 2008;16:749-56. [PubMed]
- Zhang X, Zhang L, Xu W, et al. Experimental therapy for lung cancer: umbilical cord-derived mesenchymal stem cell-mediated interleukin-24 delivery. Curr Cancer Drug Targets 2013;13:92-102. [PubMed]
- Sasportas LS, Kasmieh R, Wakimoto H, et al. Assessment of therapeutic efficacy and fate of engineered human mesenchymal stem cells for cancer therapy. Proc Natl Acad Sci U S A 2009;106:4822-7. [PubMed]
- Yan C, Yang M, Li Z, et al. Suppression of orthotopically implanted hepatocarcinoma in mice by umbilical cord-derived mesenchymal stem cells with sTRAIL gene expression driven by AFP promoter. Biomaterials 2014;35:3035-43. [PubMed]
- Yan C, Li S, Li Z, et al. Human umbilical cord mesenchymal stem cells as vehicles of CD20-specific TRAIL fusion protein delivery: a double-target therapy against non-Hodgkin's lymphoma. Mol Pharm 2013;10:142-51. [PubMed]
- Loebinger MR, Eddaoudi A, Davies D, et al. Mesenchymal stem cell delivery of TRAIL can eliminate metastatic cancer. Cancer Res 2009;69:4134-42. [PubMed]
- Uchibori R, Okada T, Ito T, et al. Retroviral vector-producing mesenchymal stem cells for targeted suicide cancer gene therapy. J Gene Med 2009;11:373-81. [PubMed]
- Kucerova L, Matuskova M, Pastorakova A, et al. Cytosine deaminase expressing human mesenchymal stem cells mediated tumour regression in melanoma bearing mice. J Gene Med 2008;10:1071-82. [PubMed]
- Kucerova L, Altanerova V, Matuskova M, et al. Adipose tissue-derived human mesenchymal stem cells mediated prodrug cancer gene therapy. Cancer Res 2007;67:6304-13. [PubMed]
- Yong RL, Shinojima N, Fueyo J, et al. Human bone marrow-derived mesenchymal stem cells for intravascular delivery of oncolytic adenovirus Delta24-RGD to human gliomas. Cancer Res 2009;69:8932-40. [PubMed]
- Xia X, Ji T, Chen P, et al. Mesenchymal stem cells as carriers and amplifiers in CRAd delivery to tumors. Mol Cancer 2011;10:134. [PubMed]
- Ong HT, Federspiel MJ, Guo CM, et al. Systemically delivered measles virus-infected mesenchymal stem cells can evade host immunity to inhibit liver cancer growth. J Hepatol 2013;59:999-1006. [PubMed]
- Mader EK, Butler G, Dowdy SC, et al. Optimizing patient derived mesenchymal stem cells as virus carriers for a phase I clinical trial in ovarian cancer. J Transl Med 2013;11:20. [PubMed]
- Li L, Guan Y, Liu H, et al. Silica nanorattle-doxorubicin-anchored mesenchymal stem cells for tumor-tropic therapy. ACS Nano 2011;5:7462-70. [PubMed]
- Sadhukha T, O'Brien TD, Prabha S. Nano-engineered mesenchymal stem cells as targeted therapeutic carriers. J Control Release 2014;196:243-51. [PubMed]
- Qiao L, Xu ZL, Zhao TJ, et al. Dkk-1 secreted by mesenchymal stem cells inhibits growth of breast cancer cells via depression of Wnt signalling. Cancer Lett 2008;269:67-77. [PubMed]
- Karnoub AE, Dash AB, Vo AP, et al. Mesenchymal stem cells within tumour stroma promote breast cancer metastasis. Nature 2007;449:557-63. [PubMed]
- Ling W, Zhang J, Yuan Z, et al. Mesenchymal stem cells use IDO to regulate immunity in tumor microenvironment. Cancer Res 2014;74:1576-87. [PubMed]
- Kyriakou CA, Yong KL, Benjamin R, et al. Human mesenchymal stem cells (hMSCs) expressing truncated soluble vascular endothelial growth factor receptor (tsFlk-1) following lentiviral-mediated gene transfer inhibit growth of Burkitt's lymphoma in a murine model. J Gene Med 2006;8:253-64.
- Martinez-Quintanilla J, Bhere D, Heidari P, et al. Therapeutic efficacy and fate of bimodal engineered stem cells in malignant brain tumors. Stem Cells 2013;31:1706-14. [PubMed]
- Ramos CA, Asgari Z, Liu E, et al. An inducible caspase 9 suicide gene to improve the safety of mesenchymal stromal cell therapies. Stem Cells 2010;28:1107-15. [PubMed]
Cite this article as: Li Z, Fan D, Xiong D. Mesenchymal stem cells as delivery vectors for anti-tumor therapy. Stem Cell Investig 2015;2:6.